Innovative 3D Printing: Emulating Human Functionality in Brain Tissue and Other Organs
- Larry Jones
- Mar 4, 2024
- 5 min read
Updated: Jun 7, 2024
The lack of dependable human neural tissues that can be used for assessing neural circuit functionality presents an impediment to the investigation of human neural network operations. In response, a 3D bioprinting system has been created to construct tissues incorporating specific human neural cell types in desired dimensions, employing a commercial bioprinter for the task.
Utilizing bioinks and advanced printing technologies, 3D bioprinting technology constructs intricate structures. The process hinges on computer-aided design models that generate patient-derived images, faithfully replicating tissue geometries of interest. Within the printing of bioinks, a combination of cells, bioactive molecules, or biomaterials is incorporated to produce personalized scaffolds. Upon printing, the neuronal progenitors undergo differentiation into neurons and subsequently establish functional neural circuits within tissue layers, both internally and across layers.
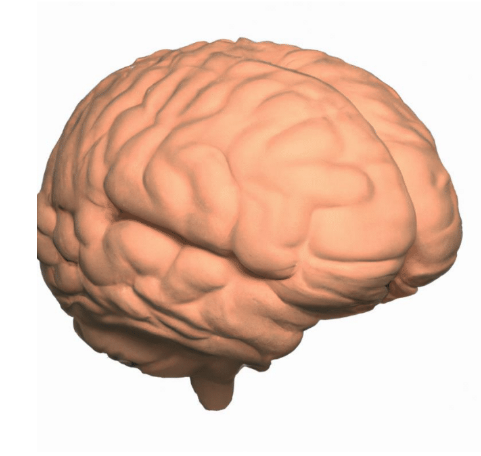
For example, printed astrocyte progenitors mature into fully developed astrocytes with complex branches. These mature astrocytes form functional networks with neurons, as shown by calcium activity and glutamate absorption when neurons are stimulated, in both normal and pathological scenarios. These engineered human neural tissues have great potential for improving our understanding of neural network connections in humans, modeling diseases, and serving as drug testing platforms.
The versatility of 3D bioprinting extends to the creation of functional living tissues and structures, including organs. It is feasible to employ bioprinting for the production of skin grafts, bone bandages, and corresponding patches for various native tissues, like the lungs.
Potential applications of 3D bioprinting encompass the following areas:
Regenerative Medicine: By allowing precise and controlled deposition of cells, hormones, drugs, and growth factors, 3D bioprinting contributes to enhancing tissue regeneration. In this context, 3D bioprinting serves as a remarkable tool due to its capacity for precise and controlled placement of essential components such as cells, hormones, drugs, and growth factors. This precision allows for the deliberate construction of complex tissue structures, facilitating the regeneration of damaged or impaired tissues within the human body.
By strategically depositing specific cell types and bioactive molecules in a controlled manner, 3D bioprinting enables the creation of personalized scaffolds that can aid in the restoration and repair of tissues. This level of precision ensures that the regenerated tissue closely mimics the natural structures, optimizing its functionality and compatibility within the patient's body.
Regenerative medicine harnesses the potential of 3D bioprinting to revolutionize the treatment of various medical conditions, offering hope for patients with injuries, degenerative diseases, and organ failure by providing a pathway towards tissue regeneration and functional recovery.
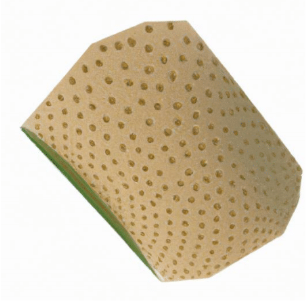
Wound Healing: This innovative technology has the capacity to play a pivotal role in accelerating the healing process for a wide spectrum of tissue injuries and wounds.
Traditionally, wound healing can be a complex and time-consuming process, particularly when it involves diverse tissue types or extensive injuries. However, 3D bioprinting introduces a new dimension to this challenge. It can be employed to create specialized biomaterials and structures that are tailored to the specific needs of the wounded area.
By precisely depositing cells, growth factors, and other bioactive components, 3D bioprinting enables the creation of custom-made scaffolds. These scaffolds provide a conducive environment for the body's natural healing mechanisms to operate more efficiently. They can support the regeneration of skin, muscle, and even bone, ensuring that the healing process is not only accelerated but also results in tissues that closely resemble the original, minimizing scarring and functional impairments.
This potential for wound healing extends to a broad range of applications, from minor cuts and abrasions to more complex injuries and surgical procedures. 3D bioprinting offers a promising avenue to improve the quality of life for individuals recovering from various wounds and injuries, allowing for faster healing and better outcomes across diverse tissue types.
Tissue Models: 3D bioprinting facilitates the creation of tissue models for the examination of infection mechanisms and the impacts of therapeutic agents. For example, Carnegie Mellon has made significant contributions to the field of 3D bioprinting. This technology enables the fabrication of complex tissue structures by layering bioinks containing cells and bioactive materials. This has promising implications for regenerative medicine as well as drug testing and development. By leveraging advancements in 3D tissue engineering and organ-on-a-chip technology, researchers are developing patient-specific disease models. These models can be used to tailor treatments and interventions to individual patients, potentially leading to more effective therapies and fewer adverse effects.
Some other examples of 3D bioprinted tissue models for studying viral infections and therapeutics are:
Brain models: A group of scientists from the University of Wisconsin-Madison has achieved a remarkable breakthrough by creating the initial instance of 3D-printed brain tissue capable of both growth and functioning in a manner resembling that of standard brain tissue. This pioneering achievement in the realm of neuroscience has catapulted research into neurological and neurodevelopmental disorders forward. The novel printing technique empowers scientists to exercise precise control over cell types and their arrangements, surpassing the capabilities of conventional brain organoids.
This innovative technique offers a versatile platform for the exploration of various neurological conditions and treatment modalities. Remarkably, it eliminates the need for specialized equipment or complex cultural methods typically associated with similar endeavors.

Courtesy: Jordan Miller/Rice University
Lung models: The lung is one of the main targets of respiratory viruses, such as influenza, SARS-CoV-2, and RSV. 3D bioprinted lung models can mimic the alveolar structure and function of the lung, as well as the air-blood barrier and the immune response. 3D bioprinted lung models can be used to investigate viral entry, replication, and spread, as well as host defense mechanisms and inflammation. 3D-bioprinted lung models can also be used to assess the efficacy and safety of antiviral drugs, vaccines, and inhalation therapies.
Liver models: The liver is a vital organ that engages in metabolism, detoxification, and immunity. The liver is also susceptible to viral infections, such as hepatitis B, C, and E, which can cause liver damage, cirrhosis, and cancer. 3D bioprinted liver models can reproduce the hepatic architecture and function, as well as the interactions between hepatocytes, stellate cells, Kupffer cells, and endothelial cells. 3D bioprinted liver models can be used to study the viral life cycle, pathogenesis, and immune response, as well as the pharmacokinetics and pharmacodynamics of antiviral drugs and vaccines.
Therapeutic Strategies: Complex 3D neuronal tissue models, replete with specific cell types and environmental cues, can be produced using 3D bioprinting to gain insights into the pathological facets and regenerative mechanisms of the nervous system.
3D bioprinting can fabricate drug delivery systems that can control the release, distribution, and targeting of therapeutic agents. 3D bioprinting can also create tissue models that can mimic the human body and its response to drugs, enabling more accurate and efficient drug screening and development. Examples of drug delivery systems and tissue models that have been 3D bioprinted include microcapsules, microneedles, scaffolds, organoids, and organ-on-a-chip.
In conclusion, 3D bioprinted tissue models can better recapitulate the complex microenvironment of the human body, such as cellular heterogeneity, extracellular matrix composition, vascularization, and mechanical properties. These factors are crucial for the interaction between pathogens and host cells, as well as the response to drugs and vaccines. 3D bioprinted tissue models can also be customized to match the patient’s specific characteristics, such as genetic background, immune status, and disease stage, enabling personalized medicine and precision diagnostics.
3D bioprinting can produce multiple tissue models with high reproducibility and consistency, allowing for the screening of a large number of infection scenarios and therapeutic agents in a short time. This methodology can also generate tissue models of different sizes and shapes, ranging from microscale organoids to macroscale organs, depending on the research and clinical needs. 3D bioprinted tissue models can reduce the reliance on animal models, which are often costly, time-consuming, and ethically controversial. Animal models also have limited predictive value for human diseases, especially viral infections, due to the differences in physiology, immunity, and susceptibility. 3D bioprinted tissue models can provide more accurate and relevant data for human health, as well as save resources and improve efficiency.
Written By: Lawrence D. Jones, Ph.D.
Comments